What Biodiversity Can Measure
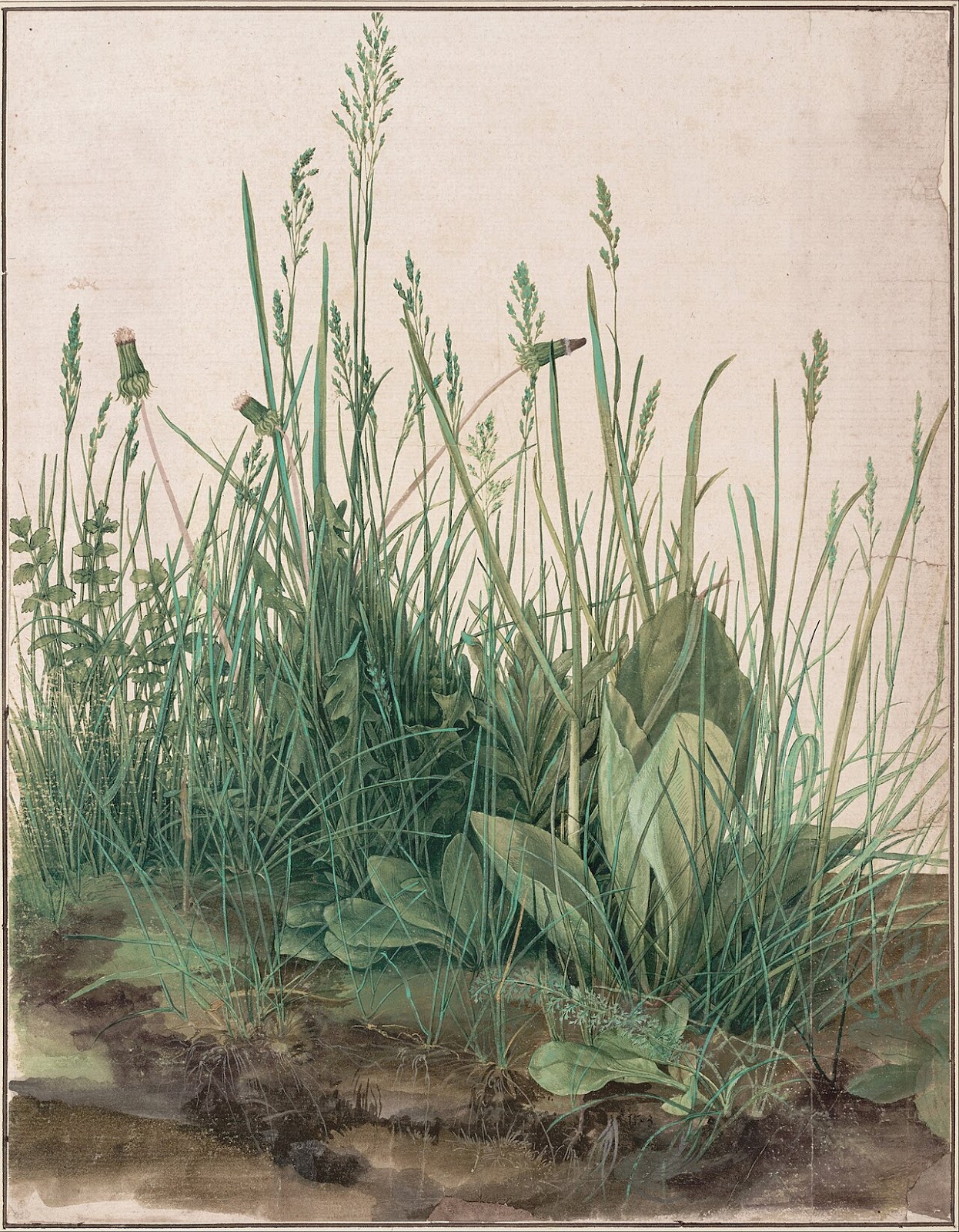
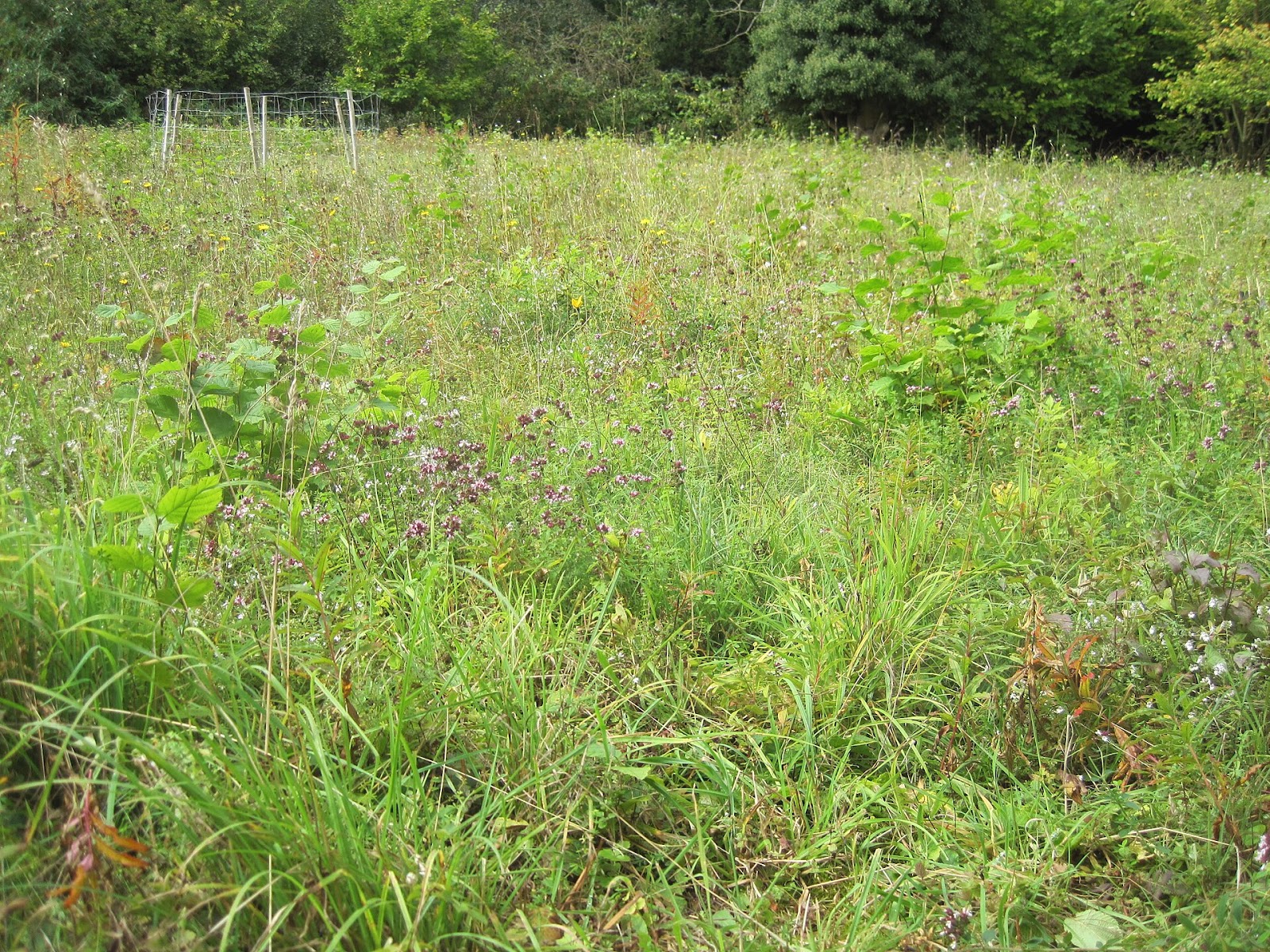
It is interesting to contemplate a tangled bank, clothed with many plants of many kinds, with birds singing on the bushes, with various insects flitting about, and with worms crawling through the damp earth, and to reflect that these elaborately constructed forms, so different from each other, and dependent upon each other in so complex a manner, have all been produced by laws acting around us. These laws, taken in the largest sense, being Growth with reproduction; Inheritance which is almost implied by reproduction; Variability from the indirect and direct action of the conditions of life, and from use and disuse; a Ratio of Increase so high as to lead to a Struggle for Life, and as a consequence to Natural Selection, entailing Divergence of Character and the Extinction of less improved forms. Thus, from the war of nature, from famine and death, the most exalted object which we are capable of conceiving, namely, the production of the higher animals, directly follows. There is grandeur in this view of life, with its several powers, having been originally breathed by the Creator into a few forms or into one; and that, whilst this planet has gone circling on according to the fixed law of gravity, from so simple a beginning endless forms most beautiful and most wonderful have been, and are being evolved. -Charles Darwin, On the Origin of Species, 1859 (final paragraph)
A path forward for quantitative biodiversity credits
Biodiversity is correctly characterized as a property of an ecological system that it is good to maximize and protect. However, it is often ill-defined, both for purposes of measurement and of showing demonstrable quantitative benefit. In the face of rapid biodiversity loss, having a meaningful measurement of biodiversity can facilitate and incentivize methods of protection and enhancement. To generate this measurement we must understand the foundational basis of such a term — both empirically and conceptually.
Humans are most familiar with defining benefit in intentional terms. The benefit is in a direction, for an extrinsic purpose. In a forest ecosystem in the process of restoration, the benefits we experience, such as reduction of erosion, replenishment of soil carbon, and the establishment of a diverse and thriving forest on previously degraded land, are certainly extrinsic in the sense that they produce beneficial consequences for us (such as improved water quality and carbon drawdown), but they are produced by the improved functioning of the ecosystem itself.
A number of interesting questions arise here. Because biodiversity has been framed since the establishment of the concept as a good in itself, less attention has been given to quantifying the benefits, or of describing the concept in relational, rather than intrinsic terms. This has also led to a conflation of biodiversity with a bald measure of the number of species present over a given area of habitat. This measurement has historically been heavily weighted toward animals and plants, with microbial and fungal diversity far less well characterized.
But we live in a relational world, where the complexity of energetic flux in a living system, be that a cell, an organism, or an ecological community, both determines the resilience of that system to external impacts, and also determines the degree of communication between the parts of that system. Framing benefit in terms of resilience to disturbance and communication between the parts of an ecosystem enables us to quantify the impact of biodiversity gain and loss, and also measure biodiversity itself in a way that correlates to quantitative impacts.
A quantitative measure of biodiversity should include these measures of energetic flux and functional connectivity, to demonstrate the benefits accrued to the system and clearly show the consequences of biodiversity loss.
So how do we convert a list of species present in an ecosystem, derived from any number of measurement methods, but easier now using technologies such as eDNA, into a metric that includes information on how these species quantitatively benefit the system as a whole?
It starts with clearing our lenses of perception. We know that the outputs of a given system are usually inputs for any number of additional systems. However, due to limits on our conception of the whole, we tend to place hard boundaries at the edges of our conceptions of systems or processes, which also interfere with our ability to see how these systems are interconnected. This habit of mind leads us to incorrectly conceive of systems in isolation from one another, when in reality the degree of functional connectivity between them is a variable like any other.
A focus on functional connectivity will help clear our lenses when it comes to concepts of both biodiversity and natural carbon capture.
Sea urchins offer a lesson in functional connectivity – in terms of understanding, measurement, and informed intervention.
Along the California coast, the once-lush forests of giant kelp that hugged the coastline are disappearing. Sea urchins consume the holdfasts of kelp plants, and sea urchin density correlates negatively with kelp abundance, and therefore with carbon removal and biodiversity in kelp forests. Sea otters and the sunflower seastar are the main predators of urchins. When human whaling reduced the populations of many large whales, orcas shifted their diet and began to prey on otters out of necessity. Now, orca predation has reduced otter populations sufficiently that urchins have been released from predation pressure (exacerbated by a recent and ongoing outbreak of seastar wasting syndrome), and their populations along the Pacific Northwest coast have increased to the point that they consume juvenile kelp plants in the spring, before they reach the surface, greatly reducing carbon removal and resulting in the formation of depauperate expanses of exposed rock known as “urchin barrens”. Urchins are not entirely dependent on kelp as a food source, so once urchin barrens are established they tend to persist, as enough urchins remain to prevent reestablishment of kelp. There is no clearly visible connection between a reduction in large whale populations and the destruction of kelp forests, and these two species reside in different ecological networks, but they are joined by the behavioral flexibility of orcas.
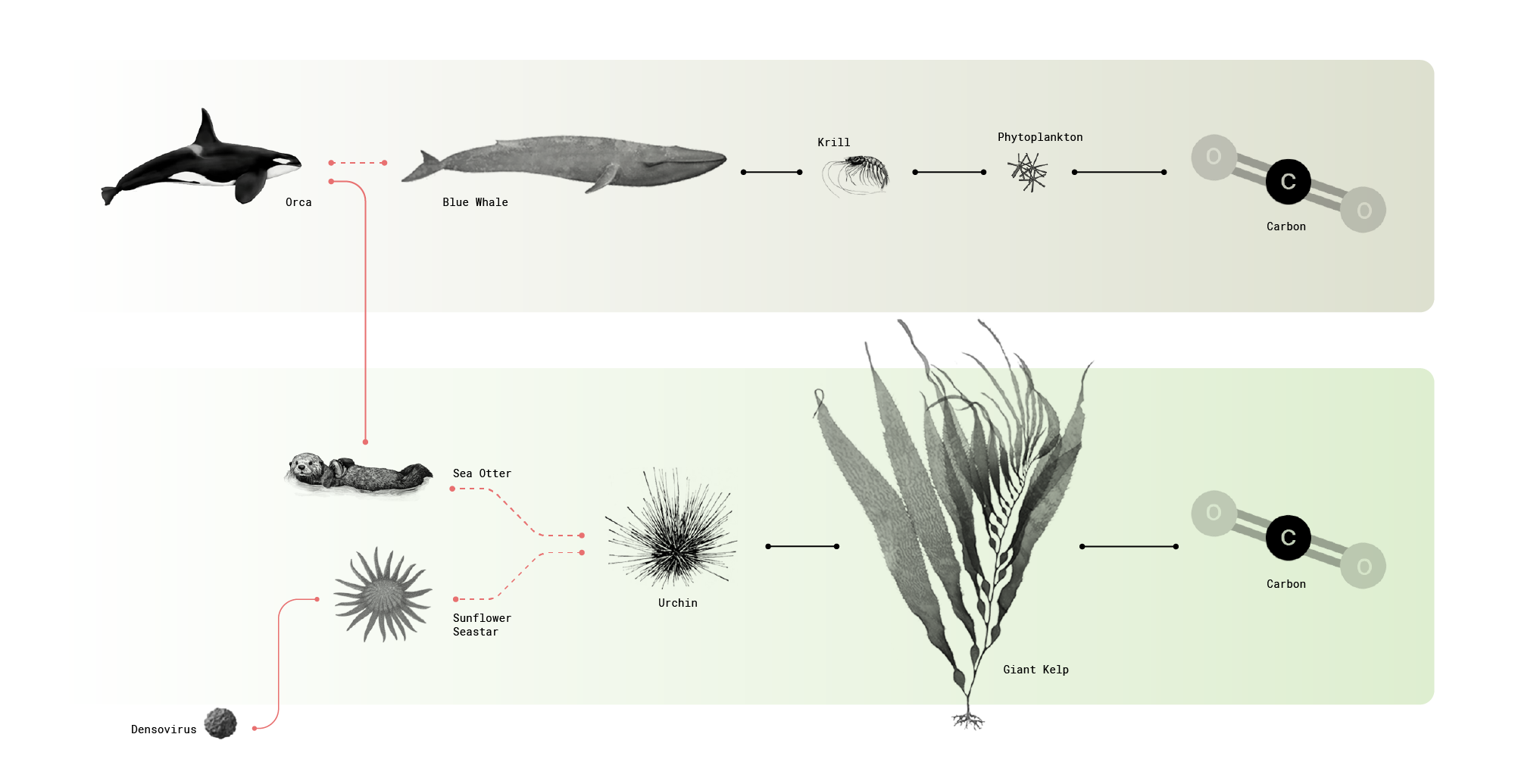
While appeal to the interconnected nature of the biosphere in justification of ecological conservation and restoration has a long history, it is not often framed in quantitative terms, as it must be if it is to be measured effectively. For the example above, this would involve calculating the energy obtained by orcas eating whales and otters respectively. Even considering this briefly, it is clear that many otters need to be consumed to replace one whale. Any ecology contains systems such as these, and the species present also differ greatly in the extent to which they contribute to functional connectivity. For example, orcas have the behavioral flexibility to seek out new prey sources, leading to a connection between open ocean and near shore environments that was previously less significant. In this case, the impact was negative (the resilience of the kelp forest ecosystem was greatly reduced by the removal of its keystone species, and the irruption of orcas was energetically “one way”, in that they removed otters without giving anything back to the system).
Highly functionally connected species often have beneficial impacts when the energy transfer is bidirectional. For example, in tropical forests, many plants produce food specifically for ants, in return for the ants both refraining from farming sap sucking insects upon them and for removing insect parasites when they find them. Here the ants are expending energy provided by the plant to ensure the ability of the plant to continue feeding them, with the added protection racket element of ensuring the production of food bodies with the implicit threat of otherwise using the plant to feed their aphid cattle. The poplar trees that we work with also participate in this system by producing nectar droplets from their leaves to feed ants. This is all well known, but what is additionally interesting here is that while a wide diversity of plant species participate, comparatively fewer species of ants do.
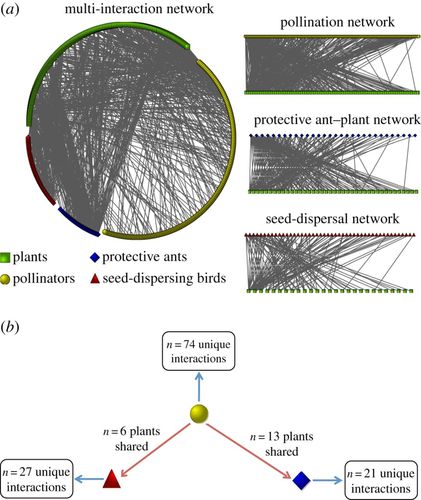
In the image above, from a study of mutualistic interactions in a forest in Veracruz, we can see that a large number of plant species (the green bar on the chart), are protected by a smaller number of ant species (the black bar). This small number of ant species is responsible for ensuring the protection of a wide diversity of plants from insect parasites. The authors of the study describe these species as ‘keystone mutualists’, recognizing that “these species and their interactions play disproportionately important roles in the community either through many direct or indirect links to other species that help guarantee the persistence of a mutualistic community rich in species.”
We can easily see from this example that removing a single protective ant species from the system would likely have a larger effect on the functioning of the ecosystem than removing a single plant species, and that this can be quantified, given prior knowledge of the ecological role played by a given species of ant.
Species that contribute to the functioning of an ecology through their wide interactions with others are known as keystone mutualists, and ecosystems are often disproportionately dependent on these species for effective functioning. This differs somewhat from the traditional definition of a keystone species as one that provides the structural basis and primary input energy for an ecology (like kelp), or is responsible for centering energetic flux through end-point predation (like orcas). Poplars, on the other hand, are keystone mutualists due to their ecological importance for other species. Populus tremula, a parent of our hybrid poplar, has more host-specific species than any other boreal tree.
How to Map Keystone Mutualists
Identifying keystone mutualists requires a relational understanding of ecology, and a model of ecosystem functioning focused on energetics. Data collection must be multimodal, and the relationships explicated may also have a degree of site-specificity.
Researchers in California have recently demonstrated the potential of merging on-the-ground observations, eDNA data, remote sensing and ecological modeling to assess community-environment interactions and ultimately map community turnover. What this specific study does well is it recognizes the necessity of representing the relationships between species present and the very different degrees to which a given species contributes toward both the resilience of the system and the communication of energy and information within the system. This frame will prove a vital foundation for market-based biodiversity banks and registries.
Human Intervention to Achieve a Quantifiable Benefit
Once we understand how to measure the health of an ecosystem in terms of keystone mutualists and measures of functional connectivity, then we can begin to intervene in a system in a quantifiable and beneficial way.
To understand how we can do this, let’s visit our examples with ants and urchins.
While in the case of protective ants, conservation of the ants to ensure functioning of the ecosystem fits easily into a classical understanding of applied ecology, the case of the urchins is somewhat different, as urchin barrens, once established, are a persistent stable state for what was previously a kelp forest. Similarly to the transformation of logged forest into savannah, or bleached coral reefs into algae pasture, they represent a phase transition in the system. They are also a species native to the area. While at the largest scale, the presence of urchin barrens is a consequence of industrial whaling, it is not a realistic expectation that the way to restore kelp forests is to restore the population of large whales, given the extremely slow population growth rate of whales. What has proven effective might just be the simplest possible strategy: direct human removal of the urchins. This action reliably results in the return of kelp forests to an area, but in the absence of otter population increase, the system remains dependent on ongoing human intervention. Kelp forest recovery has been most impressive in areas that do not have catch limits on urchins, and volunteers often simply kill the urchins by smashing them with hammers and allowing their nutrients to return to the system. In Bodega Bay in Northern California, urchins are taken from the seabed and put into aquaculture tanks where they are fed until being large enough to be used as a human food source (their eggs are extracted and form the food uni).
In these cases, human intervention to achieve a quantifiable benefit, for a specific purpose, is not synonymous with an extractive use. It is possible for correctly calibrated human interventions to both increase the functioning of an ecosystem and to generate outcomes that are beneficial to larger scale processes, such as carbon drawdown. Often, there is a choice between focusing all efforts on an ultimate restorative goal (for example the replenishment of large whales), and taking interim steps that improve the functioning of parts of a system.
How Increasing Biodiversity and Removing Carbon Can Go Hand in Hand
On the largest scale, the goals of Living Carbon are to restore functional ecological communities to land previously degraded by human extractive use, and to increase the carbon drawdown rate of trees beyond its previous value, while slowing their rate of decomposition. The first goal is of course restorative, but this is restoration with a purpose. We believe that effectively functionally connected ecologies are better at carbon sequestration than monocultures, and that increases in standing biomass and carbon retention within these ecological networks are best achieved through ensuring effective connectivity. Because of this, when we plant our trees on a site, we do this with the intent of measuring and quantifying the effect on biodiversity, so that we can learn how to best improve it.
Like the carbon markets, the measurement of biodiversity as a financial instrument is still in its infancy. The several existing registries and accreditation bodies are still settling on frameworks for how to ascribe financial value to biodiversity as an indicator of ecological functioning. We believe that the points explored above need to be considered in this process. Specifically that:
- The market value ascribed to a biodiversity credit must map to the extent to which whatever process is measured by it increases the functional connectivity of the relevant ecosystem. and
- The ultimate benefit of conserving and restoring biodiversity is in reducing the vulnerability of ecosystems to drastic phase changes as a result of external shocks or progressive internal fragmentation of their energy transfer networks.
As we have explored above, there are ways of measuring biodiversity that map to these goals, such as eDNA measurement that weighs the significance of species present in terms of their phylogenetic diversity and functional influence on the ecology. It is also important that measurement be repeated at intervals in order to show trends and effects over time.
These points are grounded in the position that our concern should be to ensure that viable ecological networks are sustained, or re-instantiated where not present, and that this will be an ongoing process of learning and experimentation informed by our own efforts to increase climate resilience and carbon removal ability of plants, supported by ongoing data collection.
This position accepts that geographic range boundaries and climatic conditions have shifted due to anthropogenic climate change, and that this will continue over at least the next century, and that the restoration of functional ecological communities is more important than whether these communities exactly match those from previous centuries.
Current approaches to quantifying and ascribing market value to biodiversity suffer from thrash when attempting to define terms such as “equivalence”, “rarity”, and “distinctiveness”. This is a consequence of their drive to both generate a fungible financial instrument and also center their measurement on restoration of a preindustrial state. For example, the World Economic Forum notes in their Biodiversity Credits Initiative, that, for offsetting purposes, “full equivalence cannot be achieved due to the distinctiveness of all aspects of biodiversity; however, companies should strive to get close to achieving equivalence and further guidance on this from standard-setters is required. As such standards emerge, companies should follow the processes and requirements they lay out. Given the multiplicity of nature, a wide range of different measurement methodologies exist and could be considered.”
A measure of biodiversity centered in the degree to which species contribute to functional connectivity and energetic flux would suffer less from these problems, and would even lead to a more accurate carbon market with proper accounting for natural solutions. Increasing biodiversity measured in this way reduces the reversal risk of removed carbon.
A system does not have to have a high diversity of photosynthetic species to remove carbon, as evidenced by the many paleo-historical examples of massive carbon removal by small numbers of proliferating species (the Azolla fern, a few species of Carboniferous trees, Cyanobacteria), but a correct analysis must understand that these charismatic species were enabled and supported by many others, less obvious in the fossil record. To use a modern example, Sphagnum moss accounts for nearly all of the carbon fixation in peat bogs (which amounts to 30% of global soil carbon storage on only 3% of land area), but “the competitive success and productivity of this keystone genus is largely dependent on symbiotic interactions with microbial associates.”
Not only this, but other microbial mutualists are necessary to protect the Sphagnum from climatic shifts and increase the resilience of the peat bog system. A correct measurement of biodiversity in a peat bog would include all these symbionts, most of which are microscopic, and would give their presence accurate weighting, perhaps finding them more significant, energetically, than charismatic species such as insectivorous plants. Peat bogs have been said to “defy the laws of biodiversity,” but I would argue that a correct accounting of the associated microbial communities would show that they are in fact diverse, just not from a muticellular-centric perspective.
The authors of this same study also observed resilience of peat bogs to climate change. They “analyzed 560 intact peat bog samples from 56 European countries to study how peat bog ecosystems respond to different temperatures, precipitation levels and air pollution levels. They found that peat bog properties remained the same across the board, demonstrating a surprising ability to adapt to climatic variation. This is because species that were suited to a certain climate were replaced elsewhere by other species that were better suited to the new climate yet serving the same function within the ecosystem.”
This provides important empirical support for the position that increasing and maintaining the functional connectivity of ecosystems should be our primary goal in encouraging effective carbon drawdown and resilience to the effects of climate change, and that the roles and functions of species present are often more important than their exact taxonomic identity.
A Biodiversity Framework for Heavily Modified Landscapes
A biodiversity framework centered on functional connectivity is especially important when considering heavily modified landscapes. The Los Angeles River (Tongva name Paayme Paxaayt, The West River) has been transformed along most of its urban course into a concrete lined flood control channel, although portions of the bed remain unlined, enabling the growth of cottonwood trees and riverine vegetation. Scientists are beginning innovative efforts to measure biodiversity and quantify change over time, while local groups are seeking to transform adjacent brownfield sites into public parks and open spaces.
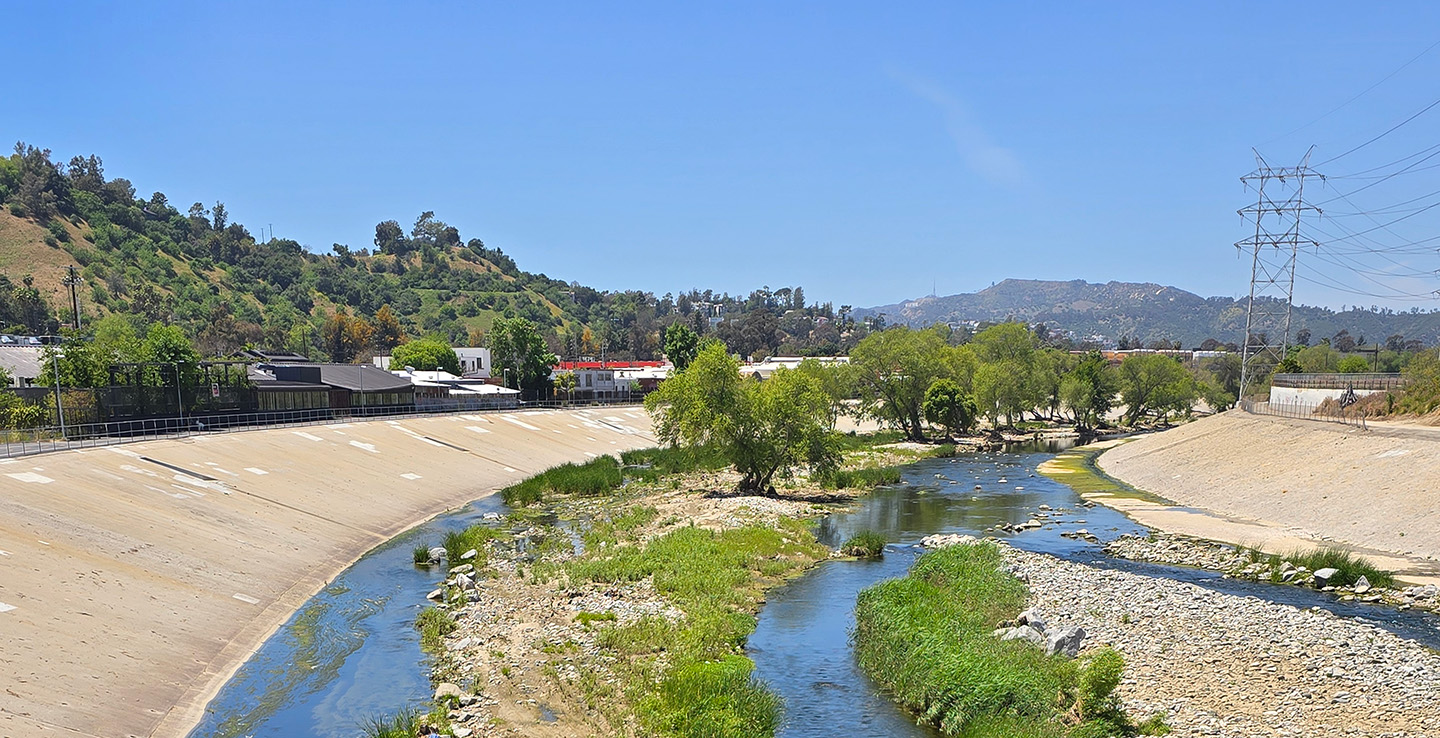
The Taylor Yard G2 River Park Project sits atop a former railroad maintenance site. Its soils are heavily contaminated with diesel fuels, and the yard is currently under the regulatory oversight of the California Department of Toxic Substances Control (DTSC).
Living Carbon successfully planted trees at this site in conditions where other trees planted could not survive, and these have formed the nucleus of a resurgent ecological community. This placement of a ketone mutualist species will restore functional connectivity and improve the state of the river itself, by absorbing and filtering pollutants from this brownfield site before they can reach the river.
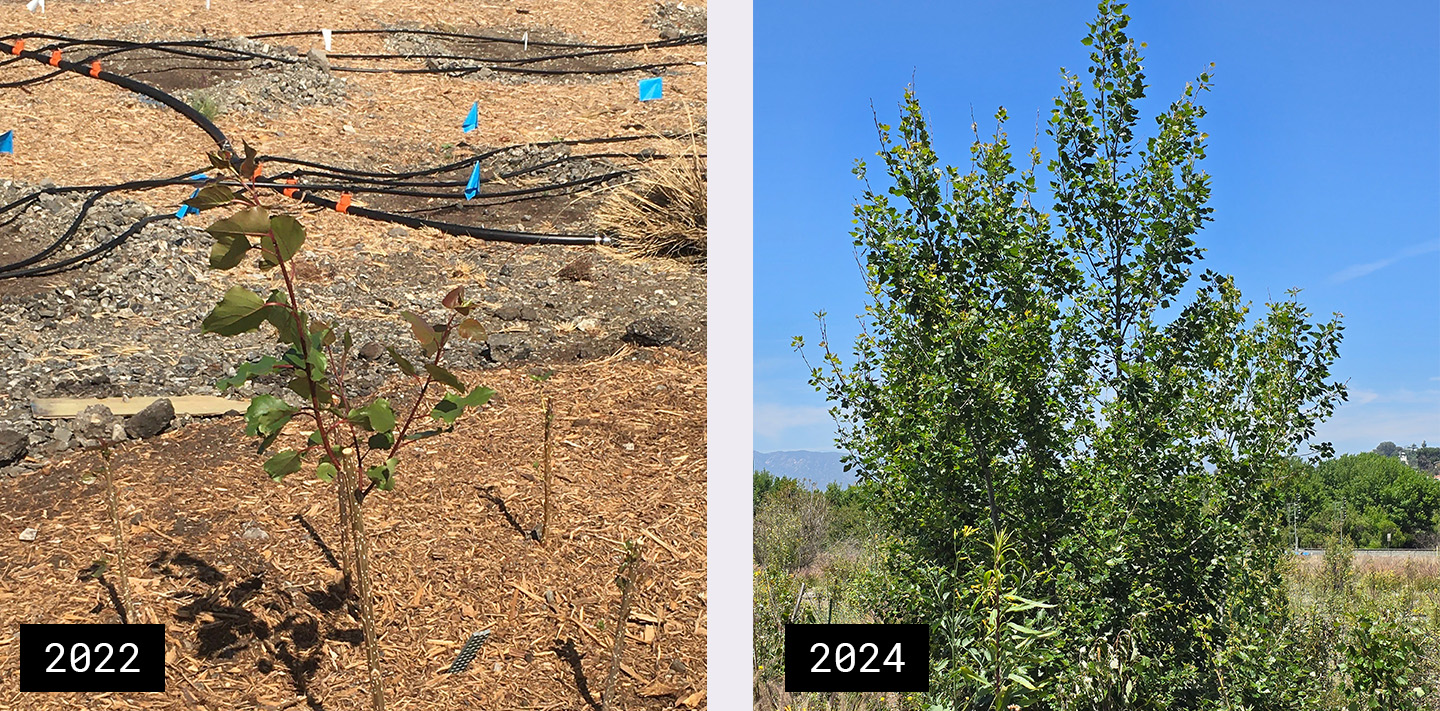
In 2022, in cooperation with Friends of the LA River and Sand County Environmental, Living Carbon provided 40 hybrid poplar saplings for an experimental planting in a portion of the yard (the orange rectangle). In March 2024, we provided an additional 24 larger trees from one of our varieties engineered for enhanced photosynthesis, drought and high temperature tolerance, and improved rate of pollutant removal. In both plantings, the asphalt underlying much of the ground was broken up, and compost and mycorrhizal fungi were added.
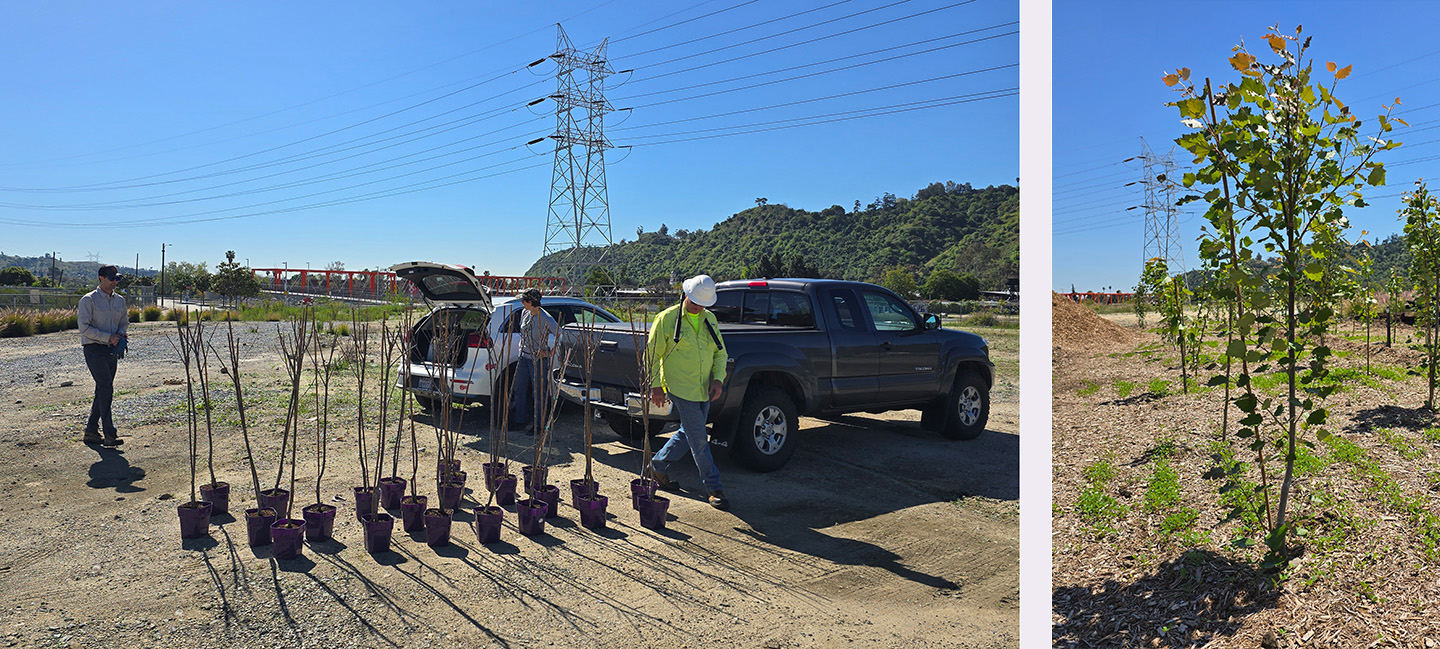

Visiting the site after two years, we saw robust growth and clear tolerance of the challenging site conditions by our original trees, with evidence of regrowth of diverse vegetation, bees, and hummingbirds perching in their branches.
We aim to remain engaged with this site and treat it as a test-bed to explore and refine best practices for increasing and measuring functional connectivity and ecological resilience while improving the state of the urban environment as the impacts of climate change continue to challenge biotic communities.
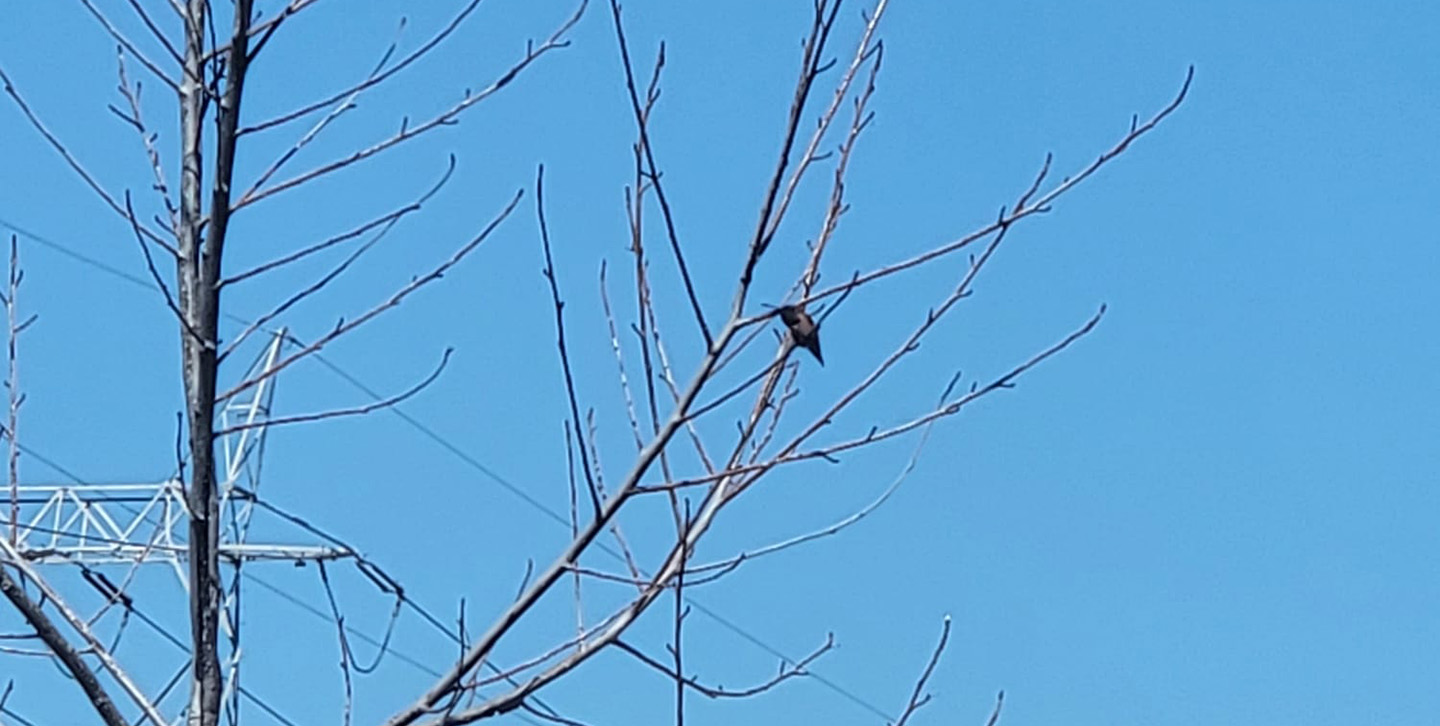
We advocate for biodiversity credits that are relevant not only to restoring pre-industrial ecosystems, but also to the necessary regeneration of degraded landscapes, such as brownfield sites, former landfills and mine lands.
We need to accept that biodiversity is 1. measurable and 2. might look different than we imagine. Biodiversity might be a cottonwood rooting through the gap in concrete, an enhanced poplar rooting in toxic soil, or the persistence of a peat bog community with different member species better suited to changing climate. Humans have a role to play, and we have methods of measurement to help guide the way.
By focusing on the flow of energy in a system, we can become clear about what we value and structure markets accordingly – incentivizing the great scope of repair that will be necessary for our future thriving as a species.