Snowball Earth I
In the thawing world after Earth’s longest ice age, we see the beginning of microbes starting to collaborate with one another for survival.
The cyanobacteria were now firmly established and, over the next 380 million years, developed full resistance to the oxygen they produced. Similar to their modern relatives, blue-green algae such as Spirulina, they held their cells together in filaments and sheets. Within these algal biofilms, cells started to specialize. Some cyanobacteria developed a new type of cell, the heterocyst, which contained the metabolism to fix nitrogen directly from the atmosphere, protected from the now omnipresent oxygen by a thick resistant wall. These algae, with the innovations of full oxygen resistance and nitrogen fixation, and even a precursor of multicellularity, finally escaped the bloom and bust cycles that limited their global impact. They could now be restrained only by the ice, which once again began to expand.
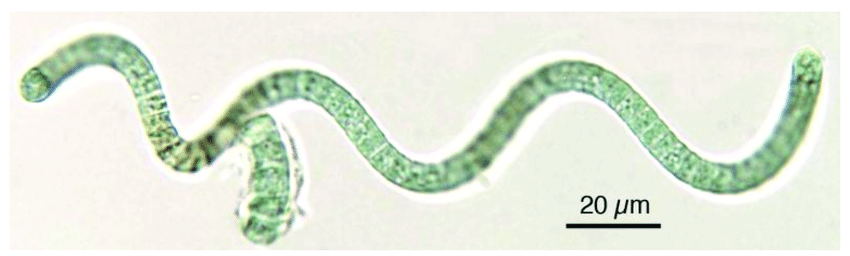
Around Lake Huron in North America, massive, ice-smoothed boulders were formed from a matrix of Early Proterozoic rock. They were dragged hundreds of miles from their origins by the world-spanning glaciers of the longest ice age the Earth has even known, the Huronian Ice Age, which reached the equator and lasted for 300 million years.

During this time, life persisted in scattered oases warmed by geothermal heat. Plate tectonics subducted organic carbon fixed by the Archean biosphere into the mantle, which was then released as CO2 during volcanic eruptions.
The core of the Earth was hotter then, and the mantle more liquid, so these eruptions often consisted of long tubes of fluid lava forcing their way through deposits of buried carbon to erupt on the surface like boiling mud. The heat and pressure of the lava transformed some of this carbon to diamond, as the bulk of it oxidized and was released as CO2 into the atmosphere. This is why most naturally occurring diamonds date from the early Proterozoic. These glittering carbon stones encrust the walls of lava tubes known as kimberlite pipes, tracing the story of how the geosphere saved the biosphere from itself. Once again, volcanic activity returns fixed carbon to the atmosphere and thaws the planet. This time, it took 300 million years for volcanoes to raise carbon dioxide levels high enough to finally melt the ice, with several pulses of full snowball Earth conditions separated by slushball interludes.
After the final thaw from the Huronian glaciation, life learned how to better exploit the massive carbon influx enabled by photosynthesis, by harnessing the reactivity of oxygen to burn sugars back to carbon dioxide gas. This drove a powerful metabolic flux that extracted many times more energy from fuel nutrients than had ever been possible before. This process, called aerobic respiration, was an innovation by organisms that were the ancestors of all living aerobic organisms - including our own mitochondria. This at last provided a balance to the photosynthetic cyanobacteria, enabling carbon to be oxidized at a rate comparable to its reduction.
The eukaryotic cell evolved at this time: a cell with internal membranes and distinct compartments, its genome contained in a membrane-bound nucleus, and the ubiquitous mitochondria, chloroplasts, and other organelles. Today’s mitochondria and chloroplasts are descendants of the earliest microbial oxidizers and reducers, now living inside another organism that shields and provides them with light and nutrients. They can optimize their provision of oxygen, sugar, and carbon dioxide. These early eukaryotes, the ancestors of modern protists, eukaryotic algae, and multicellular plants, animals and fungi, also developed sexual reproduction. By rearranging their genomes in each generation, they could counter the higher mutation rate caused by their now oxidizing environment, and accelerate their rate of evolutionary change.
During the long ice age, the oxygen had wrought yet another change on the Earth system: forming a layer of trioxygen, or ozone, in the stratosphere and reducing the extent of damaging ultraviolet radiation hitting the continental surfaces. This made survival possible in thin films of water and widened the biosphere's extent. Eventually life would be able to subsist even on dry land.
Intriguing fossils recently found in Gabon date from the period immediately following the thaw, 2.1 billion years ago in the early Proterozoic. These fossils are unprecedented in their macroscopic size and organizational complexity. They resemble the later flowering of multicellular organisms one billion years later, but likely do not have living descendents. We do not know whether they photosynthesized or fed upon other organisms, or both, but some of them may even have been capable of voluntary movement, like the later multicellular eukaryotes known as animals.
These creatures, called the Gabonionta, formed wheel-like patterns resting on the surfaces of Proterozoic river deltas, leaving suggestions of burrows in the soft mud that have now become rock. They were probably eukaryotic and certainly multicellular. At this time, oxygen levels were (for a few tens of millions of years) nearly as high as today's. These organisms likewise persisted as long as atmospheric oxygen concentrations remained high, before vanishing from the rocks, their traces replaced with black shale laid down in a lower oxygen environment.
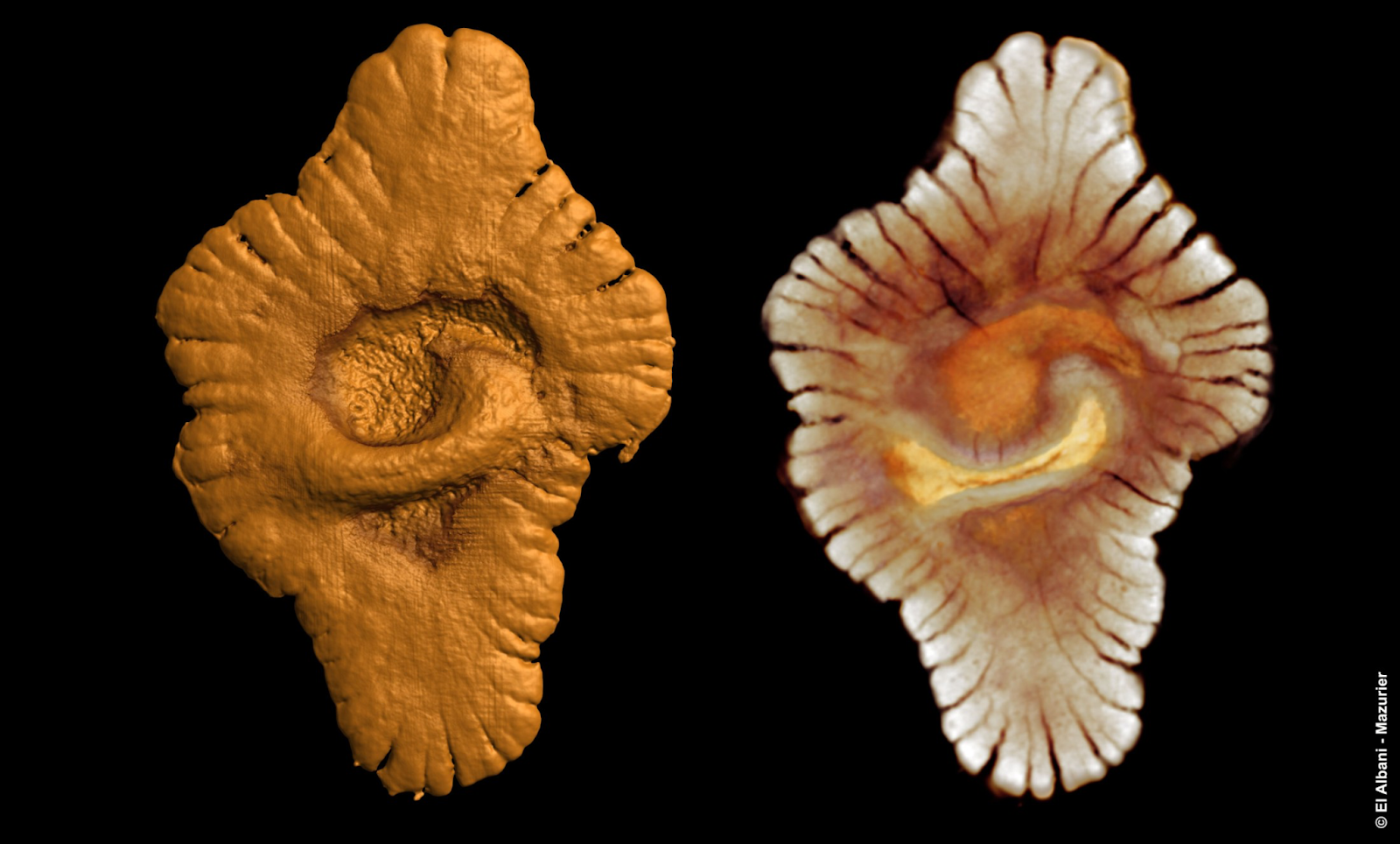
The Gabonionta’s delicate, incipient multicellularity likely came to an end due to increasingly unstable environmental conditions. The preceding Huronian ice age left much fixed carbon in the bodies of the organisms it killed. As the first aerobes evolved the capacity to decompose this biomass, they consumed oxygen and released carbon dioxide. They consumed so much oxygen that oxygen levels themselves finally fell, as aerobic respiration outpaced photosynthesis. For the first time, the oxygen that had been either a poison or a byproduct was now the lifeblood of a growing class of organisms.
But oxygen levels did not remain low for long. Over the course of the next billion years they rose steadily and inexorably, as metabolic integration tightened in the photosynthesizers. These photosynthesizers originated their own form of multicellularity in several groups of eukaryotic algae.
These were the ancestors of the modern red and green seaweeds (the brown seaweeds evolved much later from yet another layer of symbiosis). The early algae were replete with evolutionary innovations entirely beyond those of free living cyanobacteria. These cyanobacteria had now become the chloroplasts in their cells, protected, illuminated, and provided with all the CO2 they could fix. The biosphere had successfully transformed to tolerate and even depend upon the reactivity of oxygen, though it still remained vulnerable to the climatic consequences of fluctuating CO2. These complex photosynthetic collectives were now able to draw carbon dioxide from the atmosphere far faster than the mostly unicellular aerobes could return it, causing carbon dioxide to plummet toward the end of the Proterozoic, and triggering the aptly named Cryogenian (cold birth) glaciation. The Cryogenian was Earth's sharpest and deepest ice age, the ultimate Snowball Earth Event. This would be the end of the Proterozoic Eon, and the start of the next, the Phanerozoic (loosely translated as life apparent) the age of plants, animals and fungi.
This post was part of our Deep Time Series. Continue reading the next post: Snowball Earth II